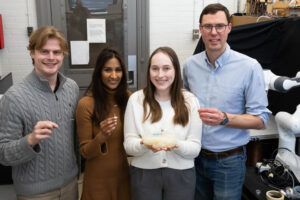
A U of T Engineering team has collaborated with researchers in the Wilfred and Joyce Posluns Centre for Image Guided Innovation and Therapeutic Intervention at The Hospital for Sick Children (SickKids) to create a set of tiny robotic tools that could enable ‘keyhole surgery’ in the brain.
In a paper published in Science Robotics, the team demonstrated the ability of these tools — only about 3 millimetres in diameter — to grip, pull and cut tissue.
Their extremely small size is made possible by the fact that they are powered not by motors but by external magnetic fields.
“In the past couple of decades, there has been this huge explosion of robotic tools that enable minimally invasive surgery, which can improve recovery times and outcomes for patients,” says Professor Eric Diller (MIE).
“We can now replicate the wrist and hand movements of a surgeon on a centimetre scale, and these tools are widely used in surgeries that take place in the torso. But when it comes to neurosurgery, we are working with an even more restrictive space.”
Current robotic surgical tools are typically driven by cables connected to electric motors, in much the same way that human fingers are manipulated by tendons in the hand that are connected to muscles in the wrist.
But Diller says that at smaller length scales, the cable-based approach starts to break down.
“The smaller you get, the harder you have to pull on the cables,” he says. “And at a certain point, you start to get problems with friction that lead to less reliable operation.”
Diller and his collaborators have been working for several years on an alternative approach. Instead of cables and pulleys, their robotic tools contain magnetically active materials that respond to external electromagnetic fields controlled by the surgical team.
The system consists of two parts. The first is the tiny tools themselves: a gripper, a scalpel and a set of forceps. The second part is what the team calls a coil table, which is a surgical table with several electromagnetic coils embedded inside.
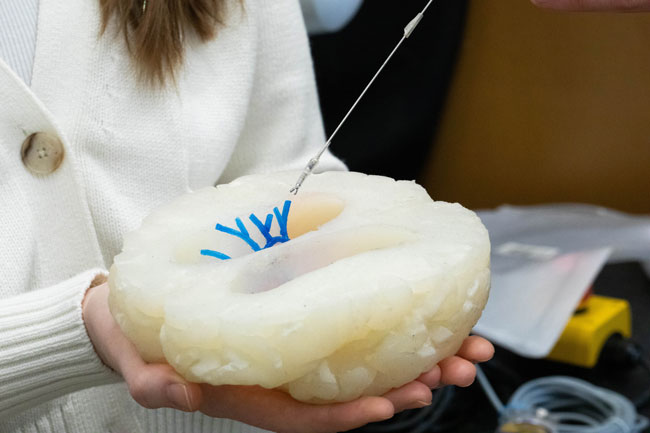
In this design, the patient would be positioned with their head on top of the embedded coils, and the robotic tools would be inserted into the brain by means of a small incision.
By altering the amount of electricity flowing into the coils, the team can manipulate the magnetic fields, causing the tools to grip, pull or cut tissue as desired.
To test the tools, Diller and his team partnered with physicians and researchers at SickKids, including Doctors James Drake and Thomas Looi. Together, they designed and built a phantom brain — a life-sized model made of silicone rubber that simulates the geometry of a real brain.
The team then used small pieces of tofu and bits of raspberries to simulate the mechanical properties of the brain tissue they would need to work with.
“The tofu is best for simulating cuts with the scalpel, because it has a consistency very similar to that of the corpus collosum, which is the part of the brain we were targeting,” says Changyan He, a former postdoctoral fellow co-supervised by both Drake and Diller, now an assistant professor at the University of Newcastle in New South Wales, Australia.
“The raspberries were used for the gripping tasks, to see if we could remove them in the way that a surgeon would remove diseased tissue.”
The performance of these magnetically-actuated tools was compared with that of standard tools handled by trained physicians.
In the paper, the team reports that the cuts made with the magnetic scalpel were consistent and narrow, with an average width of 0.3 to 0.4 millimetres.
That was even more precise than those from the traditional hand tools, which ranged from 0.6 to 2.1 millimetres.
As for the grippers, they were able to successfully pick up the target 76% of the time.
The team also tested the operation of the tools in animal models, where they found that they performed similarly well.
“I think we were all a bit surprised at just how well they performed,” says He.
“Our previous work was in very controlled environments, so we thought it might take a year or more of experimentation to get them to the point where they were comparable to human-operated tools.”
Despite the team’s success so far, Diller cautions that it may still be a long time before these tools see the inside of an operating room.
“The technology development timeline for medical devices — especially surgical robots — can be years to decades,” he says.
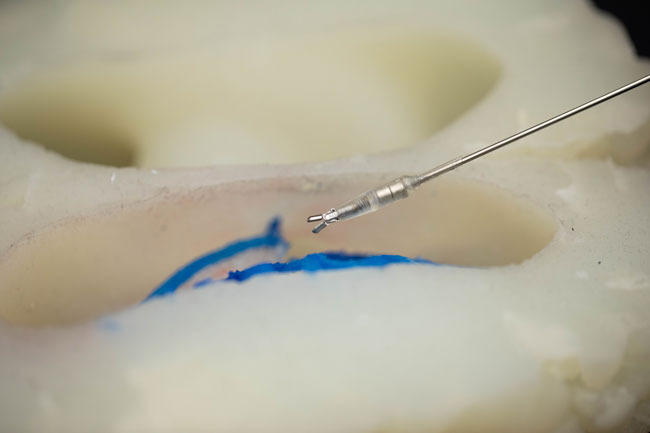
“There’s a lot we still need to figure out. We want to make sure we can fit our field generation system comfortably into the operating room, and make it compatible with imaging systems like fluoroscopy, which makes use of X-rays.”
Still, the team is excited about the potential of the technology.
“This really is a wild idea,” says Diller.
“It’s a radically different approach to how to how to make and drive these kinds of tools, but it’s also one that can lead to capabilities that are far beyond what we can do today.”
– This story was originally published on the University of Toronto’s Faculty of Applied Science and Engineering News Site on March 26, 2025, by Tyler Irving.