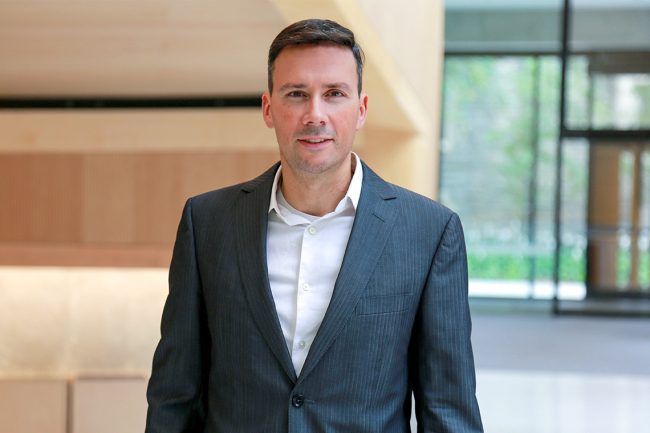
David Sinton (MIE) is the academic lead of U of T’s Climate Positive Energy Initiative, which seeks to develop clean-energy solutions by harnessing expertise across a wide range of fields. (Photo courtesy: David Sinton
Canada has formally committed to achieving net-zero greenhouse gas emissions by 2050 — and since 78% of greenhouse gas emissions globally are related to energy, finding cleaner sources is a big part of the puzzle.
Researchers at the University of Toronto have responded to this challenge by forming a new research network: the Climate Positive Energy Initiative, which seeks to harness U of T expertise, across a wide range of fields, to develop clean-energy solutions that are guided by political, human and societal considerations. It’s one of 19 projects currently supported by U of T’s Institutional Strategic Initiatives (ISI) program, a tri-campus network that unites researchers, students, faculty and external partners to fuel multidisciplinary solutions to today’s problems.
So far, the Climate Positive Energy Initiative comprises more than 100 faculty members from departments ranging from anthropology to electrical engineering. The initiative will work with community, non-profit, government and industry partners in a bid to address climate change. To enable this effort, the network aims to raise $100 million in external funding within three years.
“If you have a very narrowly defined problem, you can collect a small group of experts, execute a solution and get it done,” said Professor David Sinton (MIE), the academic lead of the new initiative, and Canada Research Chair in microfluidics and energy.
“But when you have a problem like how to respond to climate change, you’ve got an everybody problem. You have an all-profession, all-social sciences, all-natural sciences and all-humanities problem.”
“We’re united by the climate challenge.”
Sinton recently spoke to U of T News about the Climate Positive Energy Initiative, the problems it plans to tackle and what role students will play in the research network.
How did this research network come to be?
I’m trying to think if there was an apple-hitting-me-on-the-head kind of moment. I don’t think so. It was a realization that the University of Toronto has such incredible expertise — and such diversity of expertise. Great universities are unique in this regard. That’s something that struck me and others whom I was speaking to at the time.
There are many notable national labs and large corporations with climate-centred mandates — but do they have a university-sized roster of climate scientists, ethicists, economists and sustainable architecture experts? No.
That made me think: Not only is this a great opportunity, but we have a responsibility to organize around these multifaceted challenges. This is the kind of stuff that universities have to do.
Can you elaborate on that? What is the university’s role in fighting climate change?
If you have a very narrowly defined problem, then you get a very specific set of expertise and you can execute a solution and get it done. If you have a legal problem you need some lawyers. If you’ve got an engineering problem, you hire engineers and just go do it.
But when you have a problem like how to respond to climate change, you’ve got an everybody problem. You have an all-profession, all-social sciences, all-natural sciences and all-humanities problem.
Historically, we take an iterative approach toward solving problems related to energy. It’s usually tech-heavy, tech-led — and then after a decade or more we figure out what went wrong, do a cost-benefit analysis and do better next time.
We can’t do that now. The issue is time. Ten-year iterations are too slow when you have 2050 [the year by which Canada aims to reach net-zero emissions] staring at you. We have a giant multidisciplinary challenge on our hands — and a tight deadline. That’s a unique scenario.
What can we do about that? Universities can chart pathways that have some degree of consensus. We come to this challenge with a level of maturity and an understanding that no solution will be perfect. No solution will get everyone on this incredible roster of experts at U of T to align completely. But we can build a model of consensus at the University that could serve as a proxy — a way of demonstrating a pathway that was hard-fought and delivered in months instead of decades.
We also have credibility. What other organization can present a solution for de-carbonizing Canadian communities without controversy? Plans put forward by government or industry would tend to engender more skepticism. That doesn’t mean those plans are not worthy of consideration — it just means they might be less actionable.
On the other hand, a plan that comes from a university — because of its structure, academic freedom and diversity of expertise — might be more credible. We could present workable options for going forward that have a lot of traction.
De-carbonizing the Canadian North came up in a recent panel you participated in about a climate-positive energy transition. Why will this be a challenge?
We’re working on a project to bring together a national network, based at U of T, that would look at this question. The vision is to focus first on what forms of energy communities are using and what their needs are currently. What are their concerns, stated needs and opportunities for generation? Then assess if there are generalized solutions we can offer or develop together. Generalizability is important because it enables economies of scale, industry engagement and ultimate implementation.
In an energy context, we would look at what supplies they have. There are some great examples of renewable energy production in the North, but they are very seasonal — ample renewable power in the summer, followed by a shortage in winter, that means relying on fossil fuels. Saving summer’s energy for winter is the big challenge — not just in the North, but throughout Canada and much of the world.
We are engaging with local partners to tackle this seasonal energy storage conundrum and look for community-partnered solutions. Very few communities would have opportunities to store renewable electricity from summer to winter. Is it possible to store it in a chemical form compatible with their winter energy demands? We have U of T experts developing such technologies.
One last point on the North: We’re particularly interested in the Yukon grid. It’s big, isolated and they spill water, which means they have hydro dams that waste energy potential in the summer because they can’t use it and can’t store it. This renewable power capacity is lost. In the winter, they have to buy diesel and there’s all sorts of challenges with that including the logistics of getting it there, safety, energy security and dependence on southern regions.
That’s one example of a problem that requires experts across the academic spectrum — with social, political, legal, scientific and engineering backgrounds. There are opportunities to make big changes if you have those disciplines aligned.
What are some other projects or questions that researchers with the Climate Positive Energy Initiative will work on?
Closer to home, the U of T Scarborough team of researchers is looking at the transition to sustainable energy for urban communities, including Scarborough. They are studying how changes in energy use and energy systems transform the community and ecosystems. Their team is a microcosm for the whole Climate Positive Energy Initiative. They have a diversity of expertise in faculty in human geography, arts and culture, political science, management and the physical and environmental sciences.
We also have researchers exploring how the university itself can reduce its emissions and serve as an example. We’re working with Climate Positive Campus and the President’s Advisory Committee on the Environment, Climate Change, and Sustainability to lever U of T’s research expertise to reduce energy use in our buildings, enable renewable power generation, and reduce the CO2 impact of operations, heating and cooling.
The Climate Positive Campus team is hard at work and they have lots of cool projects on the go, including installing a geoexchange energy storage system under Front Campus that is projected to reduce GHG [greenhouse gas] emissions by 15,000 tonnes of CO2 equivalent by 2024.
We’re working together by putting out a call for proposals. And we are asking researchers to propose how we can do better. If the outcome of a research program could be implemented on campus within five years, we’ll co-fund the research.
Can students expect more opportunities to collaborate on research through the Climate Positive Energy Initiative?
We see student engagement as central to our mandate. Students are in everything we do. They’re absolutely critical in every activity.
We are forming a network of students from across the tri-campus with the full spectrum of expertise discussed earlier. There will also be direct support in the form of scholarships and opportunities to get involved in research projects.
We’re also aiming to engage our undergraduate students. We recognize that a lot of researchers, myself included, first became aware of the research side of the University through an undergrad thesis or project. That exposure to — and engagement in — research is super important.
Equally important is facilitating the peer environment — networks of undergraduates, graduate students and post-doctoral fellows. It’s just so enriching to work with people from diverse academic backgrounds, like those on the panel last month. You have ethicists, social scientists, scientists and engineers looking at climate and energy challenges from different vantage points. Students also benefit from this kind of multidisciplinary collaboration.
We have a great network of faculty working in this area, as we do post-docs and students. Closer collaboration through the Climate Positive Energy Initiative will give them a chance to make meaningful connections that last throughout their careers.
Consider the period between now and 2050, who’s working lifetime does that represent? Not mine. That timespan represents the professional lifetime of our current graduate students and post-doctoral fellows. This is their life. This is their challenge.
Connecting them within a diverse network of experts united by a shared challenge — that’s powerful.
Many are skeptical that we’ll meet our 2050 goals. How do you feel? Are you optimistic?
There are a few things to unpack here. I share the concern regarding the speed of change. There’s broad agreement — we need to act more quickly than we are.
In some ways we’re through with the denial phase. Most organizations and most governments are done denying climate change. We’re in the pledge phase. That’s better than the denial phase. We’re making progress.
In practical terms, the pledges are ambitious. I don’t say that to make people more depressed. It’s just really challenging. I’m not going to comment on whether Canada or other countries should have promised more or less. It’s not within my expertise. But I will tell you that all of those pledges — including the corporate net-zero pledges that are now the norm — they’re all ambitious. They all involve transformations on a scale that few appreciate.
So, it might mean — as one of our Climate Positive Energy researchers, Professor Shoshanna Saxe (CivMin), said the other day — the problem is harder than we want it to be. She’s right.
Am I hopeful? Absolutely. We’re in a real moment right now. What am I worried about? A couple of things. We’re not moving fast enough. I share that worry. What else am I worried about? That the same people who are frustrated now will get completely discouraged three years from now, 10 years from now, 20 years from now.
I feel that we need a strange combination of urgency and endurance: an urgency to get stuff done at the speed needed, and a perseverance.
That’s the challenge we’re facing. We need to have that engagement and action orientation right now, but we’re also going to need that for decades.
Where do you see the Climate Positive Energy Initiative in five or 10 years?
We need to take U of T’s climate and energy research, which is broad and diverse, put it at the forefront worldwide and enable real solutions. We will raise U of T’s already excellent research capacity in this area by an order of magnitude or two.
U of T has given us — departments and divisions across the three campuses have given us — tremendous support and they’re saying: Here’s the starting point — now get going, growing and make this a global entity.
– This story was originally published on the University of Toronto’s News Site on December 15, 2021 by Geoffrey Vendeville